Let's talk about 6G development together
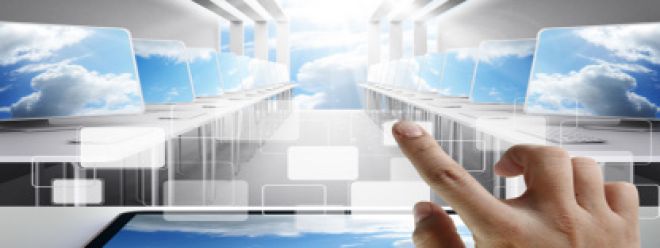
Let's talk about 6G development together
As of now, the fifth generation (5G) mobile communication system has been launched in many countries, and the number of 5G users has reached a very large scale. Now is an opportune time for academia and industry to turn their attention to the next generation. At this crossroads, an overview of the current state of technology and a vision of future communications are certainly of interest.
This paper mainly explores whether 6G is necessary under the premise that 5G is active in the field of mobile communications. Therefore, the focus of this article is to compare and discuss the technical requirements of 5G and 6G, and summarize the most advanced 6G research work and activities of some representative institutions and countries, predict the relevant specifications and standardization roadmap when 6G is produced, and briefly predict some Potentially emerging technologies and present their rationale, advantages, challenges and open research questions. This article aims to explore how 6G will change people's daily life after the birth, describe the 6G system as comprehensively as possible, and stimulate people's interest in the follow-up research and development of 6G communication systems.
introduction
The mobile communication system is the first generation of analog cellular system, namely 1G network, born in the United States and Northern Europe. Has been developed to the fifth generation. The innovation of 2G lies in the global mobile communication system GSM; the innovation of 3G lies in the revolutionary technology of Code Division Multiple Access (CDMA), represented by WCDMA, CDMA2000 and TD-SCDMA; MIMO) and Orthogonal Frequency Division Multiplexing (OFDM). 5G will expand mobile communication services from people to things, from consumers to vertical industries, from traditional mobile broadband to Industry 4.0, virtual reality (VR), Internet of Things (IoT) and autonomous driving.
Currently, 5G is still being deployed around the world, but academia and industry have shifted their attention to 6G systems to meet future information and communication needs in 2030. While there have been objections to talking about 6G[1], several pioneering works on next-generation wireless networks have already begun. In July 2018, the Standardization Sector of the International Telecommunication Union Telecommunication (ITU-T) established a focus group called "Technologies for Network 2030". The group intends to study network capabilities in 2030 and beyond [2], which are expected to support new forward-looking scenarios such as holographic communication, ubiquitous intelligence, tactile Internet, multi-sensory experiences and digital twins. European Commission launches sponsorship of research beyond 5G, as called for in Horizon 2020 - ICT-20 5G Long Term Evolution and ICT-52 Smart Connectivity Beyond 5G - a group of pioneers in key 6G technologies in early 2020 Research project launched.
The European Commission has also announced a strategy to accelerate investment in Europe's "gigabit connectivity", including 5G and 6G, to shape Europe's digital future[3]. In October 2020, the Next Generation Mobile Networks (NGMN) launched a new "6G Vision and Drivers" project, which aims to provide early and timely guidance for global 6G activities. Other traditional major players in the mobile communications field, such as the United States, China, Germany, Japan, and South Korea, have officially launched 6G research.
Key drivers for developing 6G
Since mid-2019, commercial 5G mobile networks have been rolled out globally, reaching very large scales in some countries. For example, by the end of 2020, China has deployed more than 500,000 5G base stations, serving more than 100 million 5G users. In keeping with the tradition of a new generation emerging every decade, now is the time for academia and industry to start exploring the 5G successor. However, on the road to 6G, the first question we encounter is "Is 6G really needed?", or "Is 5G already enough?", so this article discusses the key drivers for the development of 6G.
The development of next-generation systems is driven not only by the exponential growth of mobile traffic and mobile subscriptions, but also by the imminent emergence of new disruptive services and applications. Furthermore, it is driven by the inherent need of the mobile communication society to continuously improve network efficiency (i.e. cost efficiency, energy efficiency, spectrum efficiency and operational efficiency). With the advent of advanced technologies such as AI, THz and large-scale satellite constellations, communication networks can evolve towards more powerful and efficient systems to better meet the needs of current services and provide hitherto unseen disruptions. Sexual services open up possibilities.
One of the key drivers is first and foremost the explosive growth of mobile traffic. We are in an unprecedented era, with the rapid emergence and development of a large number of smart products, interactive services, and smart applications, placing huge demands on mobile communications. It is foreseeable that it will be difficult for the 5G system to adapt to the huge mobile traffic in 2030 and beyond. Due to the surge of new technology applications such as rich video applications, enhanced screen resolution, machine-to-machine (M2M) communication, and mobile cloud services, global mobile traffic will continue to grow in an explosive manner, reaching 5016 exabytes per month in 2030 , compared to 62 EB per month in 2020. A report by Ericsson [4] shows that the global mobile communication volume has reached 33 EB per month by the end of 2019, which proves the correctness of the ITU-R estimation.
Over the past decade, the number of smartphones and tablets has grown exponentially due to the proliferation of mobile broadband (MBB). This trend will continue in the 2020s as smartphone and tablet penetration is not yet saturated, especially in developing countries. At the same time, new types of user terminals such as wearable electronic devices and VR glasses are rapidly appearing on the market and quickly adopted by consumers. On the other hand, as the number of MBB users increases, the traffic demand of each MBB user continues to increase. This is largely due to the popularity of mobile video services such as Youtube, Netflix, and more recently Tik Tok, as well as the steady increase in screen resolutions on mobile devices. Two-thirds of the upcoming explosive growth of mobile traffic[4] comes from mobile video services, and mobile video services will play a more dominant role in the future. In some developed countries, rich video services drive strong traffic growth until 2025, and the long-term growth wave will continue due to the penetration of augmented reality (AR) and VR applications.
Another key driver is potential use cases. With the emergence of new technologies and the continuous development of existing technologies, such as holography, robotics, microelectronics, optoelectronics, artificial intelligence and space technology, many unprecedented applications can be cultivated in mobile networks. In order to emphasize the unique characteristics of 6G and define the technical requirements of 6G, some potential application cases of 6G are mainly listed, such as holographic communication (HTC), multi-sensory experience and mass intelligence, etc.
Holographic Communication (HTC): In contrast to conventional 3D video using binocular parallax, true holograms satisfy all visual cues for viewing 3D objects with the naked eye as naturally as possible. With the remarkable advancement of holographic display technology in recent years, such as Microsoft's HoloLens [5], its application is expected to become a reality in the next decade. Remote rendering of high-definition holograms via mobile networks will bring a truly immersive experience. For example, holographic telepresence will allow remote participants to be projected into a conference room as holograms, or allow participants in online training or education to interact with hyperreal objects. However, even with image compression, the HTC incurs enormous bandwidth requirements on the order of terabits per second.
In addition to considering frame rate, resolution, and color depth in two-dimensional (2D) video, the quality of a hologram also includes volumetric data such as tilt, angle, and position [6]. HTC also needed ultra-low latency for true immersion and high-precision synchronization across a large number of related streams to reconstruct the hologram.
Multisensory experience: Humans have five senses (sight, hearing, touch, smell, and taste) to perceive the external environment, while current communication only focuses on optical (text, images, and video) and acoustic (audio, speech, and music) media. The involvement of taste and smell can create a fully immersive experience, which may lead to some new services, for example in the food and texture industry [2]. In addition, the application of tactile communication will play a more important role and bring about a wide range of applications, such as remote surgery, remote control, and immersive gaming. This use case has stringent requirements for low latency.
Smart for the masses: With the proliferation of mobile smart devices and the emergence of new connected devices such as robots, smart cars, drones and VR glasses, aerial smart services are expected to flourish. These intelligent tasks mainly rely on traditional computing-oriented artificial intelligence techniques: computer vision, simultaneous localization and mapping (SLAM), face and speech recognition, natural language processing, motion control, etc. In order to overcome the strict computing, storage, power and privacy constraints on mobile devices, 6G networks will use AI as a service by utilizing distributed computing resources on the cloud, mobile edge and terminal devices, and cultivating ML training and jamming mechanisms for efficient communication. The way to provide general intelligence [7]. For example, humanoid robots such as Boston Dynamics' Atlas [8] can offload the computational load of SLAM to edge computing resources to improve motion accuracy, extend battery life, and become lighter by removing some embedded computing components. In addition to computationally intensive tasks, pervasive intelligence facilitates time-sensitive AI tasks to avoid the latency limitations of cloud computing when quick decisions or responses to conditions are required.
6G technical requirements
To well support disruptive use cases and applications in 2030 and beyond, 6G systems will offer extremely high capacity, reliability, efficiency, and more. Like the IMT-2020 minimum requirements specified in [9], a number of quantitative or qualitative KPIs are used to indicate the technical requirements for 6G. Most of the KPIs used to evaluate 5G are still applicable to 6G, while some new KPIs will be introduced for the evaluation of new technology characteristics.
The following 5 KPIs can be considered as key requirements in the definition of 5G, briefly introduced as follows:
Peak data rate: Driven by user demand and technological advancements such as THz communication, it is expected to reach 1Tbps, dozens of times that of 5G. The peak rate of 5G is 20 Gbps in the downlink and 10 Gbps in the uplink.
User Experience Data Rate: This value is defined as the 5th percentile (5%) of the cumulative distribution function of user throughput. In other words, users can get at least 95% of the data rate at any time or location. It is more meaningful to measure the perceived performance, especially at the cell edge, and reflect the quality of the network design (such as site density, architecture, inter-cell optimization, etc.). In a 5G deployment scenario in a dense city, the target user perception rate is 100Mbps for the downlink and 50Mbps for the uplink. 6G is expected to provide a higher 1Gbps, 10 times that of 5G.
Latency: Latency can be divided into user plane and control plane latency. The former is the time delay in a wireless network from when a packet is sent at the source to when it is received at the destination, assuming the mobile station is active. In 5G, eMBB has a minimum requirement of user plane latency of 4ms and URLLC of 1ms. Expect this value to drop further down to 100µs or even 10µs. Control plane latency refers to the transition time from the most "battery efficient" state (e.g., idle state) to the start of continuous data transfer (e.g., active state). The minimum latency of the control plane in 5G should be 10ms, and it is expected to improve significantly in 6G. Besides over-the-air latency, round-trip or E2E latency makes more sense, but is also complex due to the large number of network entities involved. In 6G, E2E latency can be considered as a whole.
Mobility: Mobility implies the maximum speed at which a mobile station can move by the network while providing acceptable quality of experience (QoE). In order to support the deployment scenario of high-speed trains, the maximum mobility supported by 5G is 500 km/h. In 6G, if commercial aviation systems are considered, the maximum speed is 1000 km/h.
Connection density is a KPI for evaluation in mMTC usage scenarios. In the case of a limited number of wireless resources, the minimum number of devices with relaxed QoS per square kilometer is 106 5G devices, which is expected to further increase by 10 times to 107 devices per square kilometer.
visible light communication
VLC operates in the frequency range of 400THz to 800THz. Unlike lower THz-range radio-frequency technologies that use antennas, VLC relies on an illumination source (especially a light-emitting diode (LED)) and an image sensor or photodiode array to implement the transceiver. Using these transceivers, high bandwidth can be easily achieved at low power consumption (100 mW at 10 Mbps to 100 Mbps) without electromagnetic or radio interference. The high energy efficiency, long lifetime (up to 10 years), and low cost of mainstream LEDs, combined with unlicensed spectrum access, make VLC a viable option for battery life and access cost-sensitive use cases such as massive IoT and wireless sensor networks ( WSN)) attractive solution. In addition, VLC also exhibits better propagation performance than RF technology in some non-terrestrial scenarios (such as aerospace and underwater), which may be an important part of the future 6G ecosystem.
MIMO gain in VLC is very fragile compared to RF, especially in indoor scenarios. This stems from the high coherence, i.e. low spatial diversity, between propagation paths. While this consistency can be reduced to some extent by using spaced LED arrays [10], MIMO-VLC is also challenged by receiver design and implementation: non-imaging receivers are extremely sensitive to their spatial alignment with the transmitter , while imaging receivers are not suitable for cost-critical use cases due to their high prices. Therefore, despite the efforts of the academic community since ten years [11], until now, no MIMO approach has been standardized as the mainstream VLC physical layer of IEEE 802.15.7. Therefore, beamforming in VLC, unlike MIMO-based RF beamforming, is achieved by special optical devices called spatial light modulators (SLMs).
Similar to millimeter wave and terahertz technologies, VLC also relies on the LOS channel because it is neither penetrating nor diffracting enough to get around common obstacles. Meanwhile, VLC systems usually require directional antennas with narrow beams due to concerns about adjacent cell interference and almost ubiquitous ambient light noise. These facts make VLC systems highly sensitive to the user's location and mobility, resulting in high demands on beam tracking.
On the other hand, this function can also play an advantage in some usage scenarios, such as higher accuracy of indoor positioning [12] and lower interference of vehicle communication [13].
Another key technical challenge facing VLC is the open and unregulated (more specifically, unregulated) access to the visible light spectrum, which means that VLC systems pose a greater security risk than traditional cellular systems in licensed RF bands. Higher, more stringent security requirements are required. Regarding this, physical layer security has been extensively studied as a promising solution [14].
Summary and Outlook
This paper provides a partial survey of the drivers, demands, dynamics and enablers of 6G mobile systems. It can be concluded that the traditional evolution of new generations every decade will not end with 5G, given the enormous enthusiasm in academia and industry for the development of 6G, the first 6G networks are expected to be deployed in 2030 or earlier. 6G will accommodate use cases and applications introduced in 5G, such as Internet of Things, Industry 4.0, virtual reality and autonomous driving, in a more cost-effective, energy- and resource-efficient manner, and provide better quality of experience. At the same time, it will enable unprecedented use cases that 5G cannot support, such as holographic communication, pervasive intelligence, global ubiquitous connectivity, and other disruptive applications that we cannot yet imagine. Starting from the introduction of MTC and IoT in the 5G era, the trend of mobile communication services has expanded from being only human-centric to connecting machines and things, and this trend will continue, and the Internet of Everything will be realized when 6G arrives. 6G systems must meet extremely stringent requirements for latency, reliability, mobility, and security, and significantly improve coverage, peak data rate, user experience rate, system capacity, and connection density, with KPIs typically increased by 10 compared to 5G to 100 times.
In 1926, engineer and inventor Nikola Tesla stated that "when wireless technology is perfectly applied, the whole earth will become one gigantic brain". When 6G arrives, this prediction will become a reality.
references
[1] FH P . Fitzek and P . Seeling, “Why we should not talk about 6G,” arXiv, Mar. 2020
[2] "A blueprint of technology, applications and market drivers towards the year 2030 and beyond," White Paper, ITU-T FG-NET-2030, May 2019
[3] "Shaping Europe's digital future," Communication-COM(2020)67, European Commission, Brussels, Belgium, Feb. 2020.
[4] "Mobile data traffic outlook," Report, Ericsson, Jun. 2020.
[5] Microsoft HoloLens. [Online]. Available: https://www.microsoft.com/en-us/hololens/
[6] A. Clemm et al., “Toward truly immersive holographic-type communication: Challenges and solutions,” IEEE Commun. Mag., vol. 58, no. 1, pp. 93–99, Jan. 2020.
[7] KB Letaief et al., “The roadmap to 6G: AI empowered wireless networks,” IEEE Commun. Mag., vol. 57, no. 8, pp. 84–90, Aug. 2019
[8] Boston dynamics. [Online]. Available: https://www.bostondynamics.com/atlas
[9] Minimum requirements related to technical performance for IMT-2020 radio interface(s), ITU-R Std. M.2410-0, Nov. 2017.
[10] A. Al-Kinani et al., “Optical wireless communication channel measurements and models,” IEEE Commun. Surveys Tuts., vol. 20, no. 3, pp. 1939–1962, 2018
[11] N. Huang et al., “Transceiver design for MIMO VLC systems with integerforcing receivers,” IEEE J. Sel. Areas Commun., vol. 36, no. 1, pp. 66–77, 2018
[12] Y . Zhuang et al., “A survey of positioning systems using visible LED lights,” IEEE Commun. Surveys Tuts., vol. 20, no. 3, pp. 1963–1988, 2018.
[13] A. Memedi and F. Dressler, “V ehicular visible light communications: A survey,” IEEE Commun. Surveys Tuts., pp. 1–1, 2020.
[14] MA Arfaoui et al., “Physical layer security for visible light communication systems: A survey,” IEEE Commun. Surveys Tuts., vol. 22, no. 3, pp. 1887–1908, 2020.
Translation modified from: W. Jiang, B. Han, MA Habibi and HD Schotten, "The Road Towards 6G: A Comprehensive Survey"